The foundation of any high-rise building lies in its ability to withstand massive loads without failure. Steel beams play a pivotal role in supporting the weight of these towering structures, ensuring they remain stable, safe, and structurally sound for decades. However, calculating the load-bearing capacity of steel beams is not just a routine engineering task—it is a critical process that determines the safety, efficiency, and longevity of a building.
For engineers, project managers, and EPCI professionals, understanding the science behind load calculations is non-negotiable. Whether you’re designing a modern skyscraper in Lagos, a commercial hub in New York, or a high-rise residential complex in Dubai, mastering load-bearing calculations is key to preventing catastrophic failures. This article provides a deep dive into the fundamental principles, advanced methodologies, and industry best practices to ensure steel beams meet global engineering standards and regulatory requirements.
Understanding Load Types and Their Impact on Steel Beams
Steel beams serve as the backbone of modern construction, providing the necessary support for high-rise buildings, bridges, and industrial structures. To ensure the safety and longevity of these structures, engineers must carefully analyze the different types of loads acting on the beams. Each load type influences the structural integrity in a unique way, and failure to account for them can lead to catastrophic consequences. By understanding how these forces interact with steel beams, construction professionals can optimize design, reduce material costs, and enhance safety.
Dead Loads: The Constant Weight of the Structure
Dead loads are the permanent, unchanging forces that a structure must bear throughout its lifespan. These include the self-weight of the building’s framework, walls, floors, ceilings, and other non-movable components. Additional elements such as HVAC systems, plumbing, electrical installations, and fixed equipment also contribute to the dead load. Because these forces remain constant over time, they provide a predictable baseline for structural calculations. Engineers must ensure that steel beams are designed to bear these loads without excessive stress or deformation. Inadequate consideration of dead loads can lead to long-term structural issues such as sagging, cracking, or material fatigue.
Live Loads: The Ever-Changing Forces in Motion
Unlike dead loads, live loads are dynamic forces that fluctuate based on usage and occupancy. These include the weight of people, furniture, vehicles, and any temporary loads introduced during daily activities. In commercial buildings, factors such as office equipment, storage loads, and machinery add to the live load calculations. Live loads vary depending on the function of the building; for instance, a warehouse designed to store heavy goods will experience significantly higher live loads than a residential home. Engineers must account for these variations to ensure that steel beams can accommodate the shifting weight distribution without structural instability.
Wind Loads: The Unseen Lateral Pressure
Wind loads exert lateral forces on a building, impacting the steel framework in unpredictable ways. The effects of wind pressure become more pronounced in high-rise buildings, where the force increases with elevation. Wind loads can create uplift, sway, and torsional stress, potentially leading to structural fatigue or failure if not adequately managed. The shape, height, and location of the building determine how wind forces interact with the steel beams. Engineers use wind tunnel testing and computational fluid dynamics simulations to assess these impacts and design structures that can resist high wind speeds without compromising stability. Proper bracing systems, moment-resisting frames, and reinforced connections help distribute wind forces effectively across the structural framework.
Seismic Loads: The Ground’s Shifting Impact
Seismic loads arise from ground movements caused by earthquakes. These forces generate sudden, intense vibrations that subject steel beams to both lateral and vertical stresses. Unlike wind loads, which are relatively predictable, seismic forces are irregular and can vary significantly in magnitude and direction. High-rise buildings in earthquake-prone regions require specialized design considerations, including flexible joints, base isolators, and energy-dissipating systems. Steel beams must be capable of absorbing and redistributing seismic energy to prevent catastrophic failure. Engineers incorporate ductile detailing in beam connections, allowing the structure to flex rather than break under extreme seismic conditions.
Impact Loads: Sudden and Unexpected Forces
Impact loads result from sudden forces applied to a structure, such as vehicular collisions, machinery vibrations, or falling objects. These loads are unpredictable and can generate high-intensity stress in localized areas. For example, in industrial settings, heavy equipment moving across a facility can create impact loads that stress steel beams beyond their standard capacity. Bridges and transportation infrastructures are particularly vulnerable to impact loads from vehicles and trains. Engineers must incorporate redundancy and additional reinforcement in structural designs to prevent localized failures that could compromise the entire system.
The Importance of Load Combinations in Steel Beam Design
In real-world applications, steel beams do not experience these loads in isolation. Instead, multiple load types act simultaneously, creating complex stress distributions that must be carefully analyzed. Engineers use advanced structural analysis software to simulate different loading scenarios and determine the most critical stress points. The combination of dead loads, live loads, wind forces, seismic movements, and impact stresses requires a holistic approach to design. Proper load factoring, material selection, and structural reinforcements ensure that steel beams can withstand diverse conditions without failure.
Understanding the different load types and their effects on steel beams is fundamental to designing safe, durable, and cost-effective structures. Dead loads provide a constant baseline, while live loads introduce variability. Wind and seismic forces create lateral stresses, and impact loads pose unpredictable risks. By integrating these considerations into structural design, engineers can develop resilient buildings capable of withstanding both everyday usage and extreme conditions. Advanced engineering techniques, computational modeling, and high-strength materials play a crucial role in ensuring that steel beams perform reliably under diverse load conditions.
In summary, a high-rise building is subjected to multiple load types that interact in complex ways, making precise calculations essential. The primary load categories include:
- Dead Load (DL): This represents the permanent, static weight of the structure, including floors, walls, ceilings, and fixed equipment. Dead load calculations are crucial because they form the baseline for structural integrity.
- Live Load (LL): Unlike dead load, live load accounts for variable factors such as people, furniture, and movable equipment. In commercial and residential buildings, live loads fluctuate, requiring safety margins to accommodate peak weight conditions.
- Wind Load (WL): High-rise buildings are particularly vulnerable to wind forces, which exert lateral pressure on the structure. Engineers must factor in regional wind speed data and design steel beams that can resist sway and torsion.
- Seismic Load (SL): In earthquake-prone regions, buildings must withstand ground accelerations. This means steel beams need to be designed with additional reinforcements to handle seismic stress without structural failure.
- Impact and Dynamic Loads: These loads arise from elevators, vehicular movement, and mechanical vibrations, necessitating precise calculations to prevent long-term fatigue and material degradation.
Neglecting any of these load types can lead to structural instability, making it imperative to apply comprehensive load-bearing calculations that account for all possible scenarios.
The Science Behind Load-Bearing Capacity Calculation
The ability of a steel beam to support weight depends on multiple factors, including material properties, cross-sectional shape, span length, and support conditions. Engineers apply principles from mechanics and structural analysis to determine the maximum load a beam can safely bear before failure. By using fundamental calculations, they ensure that steel structures remain stable, efficient, and safe under various load conditions.
Understanding the Bending Moment
One of the most critical calculations in beam design is the bending moment, which represents the internal resistance of the beam against external forces. When a load is applied to a beam, it induces bending, creating tensile stress on one side and compressive stress on the other. Engineers analyze this bending moment to predict structural performance and avoid excessive deflection or failure.
For a simply supported beam subjected to a uniformly distributed load, the maximum bending moment (M) is calculated using the equation:
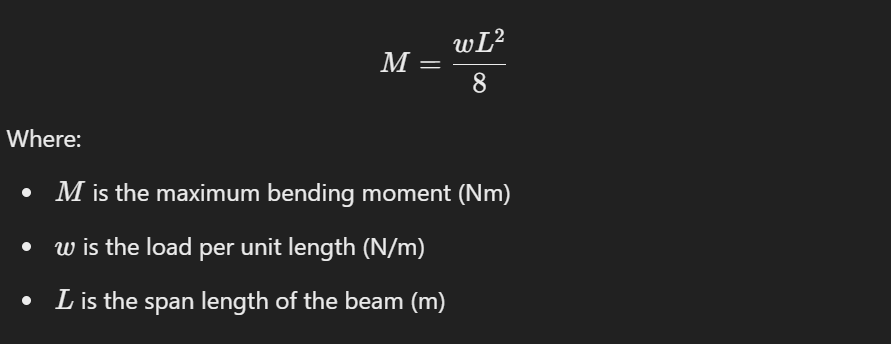
This equation provides insight into how the beam will respond to external forces. As the span length increases or as the applied load intensifies, the bending moment grows significantly, necessitating stronger beams with higher resistance to bending.
Section Modulus and Structural Strength
Once the bending moment is determined, engineers calculate the section modulus (Z) of the beam’s cross-section. The section modulus is a geometric property that directly influences the beam’s ability to resist bending. It is given by:
A higher section modulus indicates a stronger beam that can handle greater loads without excessive bending. This is why engineers carefully select beam profiles, such as I-beams, box sections, or wide-flange beams, to optimize load-bearing capacity. The choice of cross-section depends on the specific application, ensuring that the beam remains stable and efficient under various loading conditions.
The Influence of Material Properties
Material selection plays a crucial role in determining a beam’s load-bearing capacity. Steel beams are favored for their high strength-to-weight ratio, ductility, and resistance to environmental factors. The grade of steel affects its allowable stress, which is a key parameter in section modulus calculations. High-strength steel allows for slimmer, lighter beams that still provide exceptional load resistance, making them ideal for high-rise buildings, bridges, and industrial applications.
The Role of Support Conditions and Beam Span
The way a beam is supported significantly impacts its ability to carry loads. Simply supported beams, cantilever beams, and fixed beams each behave differently under applied forces. For example, a fixed beam experiences lower deflection and stress than a simply supported beam under the same loading conditions. Engineers must consider these factors when designing structural frameworks to ensure that beams perform optimally within their support configurations.
Span length also plays a vital role in load-bearing calculations. As the span increases, the bending moment rises exponentially, requiring stronger beams or additional support columns. In long-span structures like bridges and large commercial buildings, engineers often use trusses, composite materials, or reinforced steel to enhance load distribution and minimize excessive bending.
Understanding the science behind load-bearing capacity calculation is essential for designing safe and efficient steel structures. By analyzing bending moments, section modulus, material properties, and support conditions, engineers can optimize beam strength and prevent structural failures. Advanced computational tools and real-world testing further refine these calculations, ensuring that modern steel beams meet the demands of high-performance construction projects.
Factors Influencing Load-Bearing Capacity in Steel Beams
The load-bearing capacity of steel beams is a critical consideration in high-rise construction, ensuring structural stability and safety. Several factors influence how well a beam can support applied loads without excessive deflection or failure. These factors include material properties, beam geometry, span length, support conditions, serviceability requirements, and environmental considerations. By understanding these influences, engineers can optimize beam selection and design to meet the demands of modern construction projects.
Steel Grade and Yield Strength
The type and grade of steel used in a beam significantly impact its load-bearing capacity. Higher-strength steel grades, such as S355 or ASTM A992, exhibit superior yield strength and resistance to bending, making them ideal for heavy-load applications. Yield strength defines the stress level at which steel deforms permanently, and higher values allow for slimmer, more efficient beam designs while maintaining structural integrity. In contrast, lower-grade steels require thicker sections to achieve the same load resistance, increasing material costs and overall weight. Selecting the appropriate steel grade is crucial for balancing performance, safety, and cost-effectiveness in structural design.
Beam Size and Cross-Sectional Shape
The shape and size of a steel beam play a crucial role in determining its load-bearing capacity. Common beam profiles include I-beams, H-beams, and box beams, each offering unique structural advantages. I-beams and H-beams are widely used in construction due to their high moment of inertia, which enhances bending resistance. Box beams, often employed in bridge construction and heavy-load applications, provide excellent torsional rigidity. Engineers select beam profiles based on factors such as load distribution, spanning capacity, and cost efficiency. Optimizing cross-sectional geometry helps maximize strength while minimizing material usage.
Span Length and Beam Support Conditions
The length of a beam span directly affects its bending moment and deflection. Longer spans experience increased bending stresses, necessitating thicker beams or additional reinforcements. Engineers use structural analysis to determine the optimal beam size and support placement to prevent excessive deformation.
Support conditions also influence a beam’s ability to bear loads. Simply supported beams rely on external supports at both ends and are subject to higher bending moments at the center. Fixed beams, which are rigidly connected at both ends, exhibit reduced deflection and enhanced load-carrying capacity. Continuous beams distribute loads more efficiently across multiple supports, reducing peak stresses. The selection of an appropriate support system ensures that beams perform effectively under varying structural demands.
Deflection Limits and Serviceability Requirements
Structural design extends beyond just strength and stability—it also considers serviceability factors such as deflection limits. Excessive deflection in steel beams can lead to cracking in adjacent materials, misalignment of structural components, and discomfort for occupants. Design codes impose strict limits on allowable deflection to maintain both structural integrity and aesthetic quality.
Engineers use formulas to calculate deflection and ensure that beams remain within acceptable limits. Stiffness, measured by the beam’s moment of inertia and material elasticity, plays a vital role in minimizing deflection. Reinforcement techniques, such as increasing beam depth or incorporating composite materials, help enhance stiffness and reduce unwanted movement.
Environmental and Climate Considerations
In tropical regions such as Nigeria, environmental factors significantly influence the longevity and performance of steel beams. High humidity, temperature fluctuations, and exposure to corrosive elements can weaken steel over time. Corrosion, in particular, poses a major threat to structural integrity, leading to reduced load-bearing capacity and potential failure.
Engineers implement protective measures such as galvanization, epoxy coatings, or the use of corrosion-resistant steel alloys to mitigate these effects. Proper ventilation in enclosed spaces and regular maintenance inspections further help prevent deterioration. Climate-responsive design ensures that steel structures remain durable and reliable even in harsh environmental conditions.
The load-bearing capacity of steel beams is determined by multiple interrelated factors, including steel grade, beam size, span length, support conditions, serviceability requirements, and environmental influences. By carefully analyzing these elements, engineers can design efficient and resilient steel structures that withstand applied loads without failure. Advances in material science, computational modeling, and protective coatings continue to enhance the performance of steel beams, ensuring their reliability in high-rise construction and other structural applications.
Advanced Tools and Methods for Load Calculation
Modern engineering has evolved significantly, moving beyond traditional manual calculations to incorporate advanced computational tools and simulation techniques. These innovations enhance accuracy, improve efficiency, and allow for the optimization of steel beam designs in high-rise construction. By leveraging cutting-edge technologies, engineers can predict stress distribution, analyze failure points, and develop cost-effective structural solutions before construction begins.
Finite Element Analysis (FEA) for Structural Modeling
Finite Element Analysis (FEA) has become a cornerstone in structural engineering, enabling precise modeling of complex load interactions. This method divides a beam into smaller elements, allowing engineers to simulate how different forces impact the structure under various conditions. FEA helps identify areas of high stress concentration, potential points of failure, and the overall deformation behavior of steel beams. By utilizing this advanced technique, engineers can refine designs to enhance safety and efficiency while minimizing material usage.
Industry-Leading Software for Load Calculations
Structural engineers rely on specialized software to perform real-time simulations and optimize beam configurations. Some of the most widely used tools include:
STAAD.Pro – A powerful software for structural analysis and design, STAAD.Pro allows engineers to evaluate load-bearing capacity, deflection limits, and material performance. It supports multiple design codes and can simulate various load conditions, including wind and seismic forces.
ETABS – Specifically designed for high-rise buildings, ETABS provides comprehensive structural analysis, accounting for vertical and lateral loads. It enables engineers to assess frame stability, beam deflection, and load distribution efficiently.
SAP2000 – Known for its versatility, SAP2000 is used in the analysis of bridges, industrial structures, and large-scale steel frameworks. It integrates dynamic and static load assessments to ensure structures meet performance requirements under varying conditions.
ANSYS Structural – ANSYS is a high-end simulation tool that uses FEA to analyze stress, strain, and thermal effects on steel beams. It is particularly useful for identifying weak points in complex designs and optimizing beam strength while reducing unnecessary material costs.
Real-Time Simulations and Performance Validation
The integration of software tools allows engineers to conduct real-time simulations, ensuring that steel beams meet design specifications before construction begins. These simulations take into account various factors, such as load variations, environmental impacts, and long-term structural behavior. By running multiple scenarios, engineers can identify the most efficient and durable beam configurations for any given project.
The Future of Load Calculation in Structural Engineering
As technology continues to advance, artificial intelligence (AI) and machine learning are becoming integral to load calculations. AI-driven design tools can analyze vast datasets to suggest optimal beam sizes, materials, and configurations based on historical performance and real-world testing. Additionally, the use of Building Information Modeling (BIM) allows for better collaboration between engineers, architects, and contractors, ensuring seamless integration of structural elements.
The adoption of advanced tools and simulation techniques has revolutionized the way engineers calculate load-bearing capacity in steel beams. With FEA and industry-leading software, professionals can optimize designs, reduce material waste, and enhance structural safety. As AI and machine learning continue to shape the future of structural engineering, load calculations will become even more precise, leading to more efficient and resilient high-rise buildings and infrastructure projects.
Global and Nigerian Standards for Load-Bearing Calculations
Load-bearing calculations in steel structures are governed by national and international design codes that establish standardized safety benchmarks. These codes ensure that structural elements can withstand expected loads while maintaining durability and efficiency. Compliance with these regulations is essential to prevent structural failures, optimize material usage, and enhance overall safety in construction projects. Various global and Nigerian standards dictate the methodologies for load assessments, material properties, and safety factors.
American Institute of Steel Construction (AISC) Standards
The American Institute of Steel Construction (AISC) Standards provide a comprehensive framework for the design, fabrication, and erection of steel structures. AISC specifications, such as the Steel Construction Manual, outline procedures for calculating bending, shear, and axial loads. These standards emphasize Load and Resistance Factor Design (LRFD) and Allowable Strength Design (ASD), ensuring that beams can support varying load combinations while adhering to strict safety margins. AISC guidelines are widely adopted in high-rise buildings, bridges, and industrial structures across the globe.
Eurocodes (EN 1993 – Design of Steel Structures)
Eurocodes, specifically EN 1993 (Eurocode 3: Design of Steel Structures), are a set of European standards that define the design principles for steel construction. These regulations cover aspects such as material properties, structural stability, and resistance to various loads, including wind, seismic, and dynamic forces. Eurocode 3 employs limit state design principles, ensuring that steel beams meet both ultimate strength and serviceability requirements. The adaptability of Eurocodes makes them a preferred choice in numerous countries beyond Europe, including Nigeria.
Nigerian Standards for Structural Steel Design (SON/NIS 127)
In Nigeria, the Standards Organisation of Nigeria (SON) has established the Nigerian Industrial Standard (NIS 127) for structural steel design. This standard aligns with global best practices while addressing region-specific challenges such as high humidity, corrosion risks, and varying material availability. NIS 127 incorporates aspects of BS 5950, Eurocode 3, and AISC, providing local engineers with guidelines tailored to Nigerian construction conditions. Compliance with NIS 127 is essential for ensuring that steel structures can withstand environmental factors and meet safety regulations within the country.
British Standards (BS 5950 & BS EN 1993)
The British Standards (BS 5950) and BS EN 1993 have historically played a key role in structural steel design, particularly in regions with British engineering influence, such as Nigeria. BS 5950 outlines traditional steelwork design methods, including load-bearing calculations, material selection, and structural analysis. Meanwhile, BS EN 1993 aligns closely with Eurocode 3, providing updated methodologies for modern steel construction. These standards emphasize structural integrity, ensuring that beams maintain stability under extreme conditions.
The Importance of Standardization in Load Calculations
Standardized load-bearing calculations ensure uniformity in structural design, enabling engineers to predict how steel beams will perform under real-world conditions. These standards dictate:
- Material Properties: Ensuring that steel beams meet minimum strength and durability requirements.
- Safety Factors: Providing margins of safety to accommodate unforeseen variations in loading conditions.
- Load Combination Methodologies: Defining how different loads (dead, live, wind, seismic) interact and impact steel structures.
Adhering to these regulations guarantees the resilience of buildings, bridges, and industrial structures, minimizing risks associated with load-induced failures.
Global and Nigerian standards for load-bearing calculations serve as critical guidelines for structural engineers, ensuring that steel beams are designed to withstand applied forces without failure. Whether following AISC, Eurocodes, SON/NIS 127, or British Standards, compliance with these frameworks enhances safety, durability, and efficiency in construction projects. As Nigeria continues to expand its infrastructure and high-rise developments, adherence to these standards will be vital in maintaining world-class engineering practices and structural reliability.
Why EPCI Professionals Must Stay Updated
Engineering is constantly evolving, with new technologies, materials, and methodologies reshaping the way load-bearing calculations are performed. Staying informed about the latest innovations—such as ultra-high-performance steel, AI-driven predictive analysis, and 3D-printed structural elements—can give engineers a competitive edge.
Investing in continued professional development, attending industry conferences, and leveraging platforms like EPCI.ng ensures that engineers remain at the forefront of industry advancements.
Conclusion
The integrity of a high-rise building depends on precise and well-researched load-bearing calculations for steel beams. A small miscalculation can lead to disastrous consequences, including structural failure and financial losses. Whether you’re an established engineering professional or an aspiring student, mastering these principles is crucial to ensuring the safety, efficiency, and success of any high-rise project.
Take your engineering expertise to the next level! Subscribe to EPCI.ng today for in-depth engineering insights, cutting-edge industry trends, and expert knowledge that will keep you ahead in the field. For more Updates, follow us on X (Fomerly Twitter), Instagram, LinkedIn, Facebook